This web page was produced as an assignment for Genetics 677, an undergraduate course in UW-Madison
Mice – GSK3B – a central regulator of embryonic myocyte proliferation
As previously discussed (see primary article review) Kerkela and colleagues [2] performed genetic studies in mice where they created knock out (deletion of the whole gene) mutant mice which were lacking either GSK3α or GSK3β gene. According to the researchers mice lacking GSK3α were able to survive, were fertile and were born in normal Mendelian ratios, in contrast no life embryos were recovered in mice lacking GSK3β(GSK3b-/-). The GSK3b-/- embryos showed ventricular septal defects, double outlets of the right ventricle (RV) and hypertrophy o the cardiac tissue where the ventricular cavities were almost completely obliterated (Figure 1 and 2). The scientists were able to demonstrate that the expression of GATA4, cyclin D1 and c-Myc was increased – those three factors are key regulators of proliferation. Altogether the study was able to demonstrate that GSK3b and not GSK3a is necessary for the normal heart development. In addition the study demonstrated that there is no changes of β-catenin localization in the three different phenotypes (WT, GSK3b-/- and GSK3a-/-), which suggests redundancy in the β-catenin regulation by the two isoforms.
Figure 1
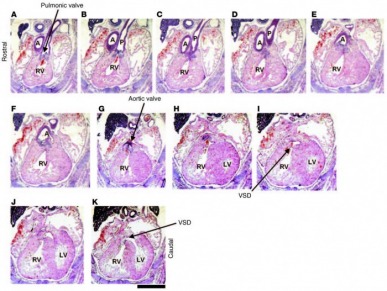
Figure 1 Histological analysis of heart development in GSK-3β–deficient mice. Sequential rostral-to-caudal sections from an E15.5 Gsk3b–/– embryo from the pulmonary valve (top left) to the ventricles (bottom right) (original magnification, ×2.0; scale bar: 0.5 cm). The pulmonary valve and the pulmonary artery (P) are positioned over the RV (A–C). Below the pulmonary valve, the aorta (A) descends toward the aortic valve (D–F); the aortic valve is positioned over the RV (G). Caudal to the semilunar valves, the RV and the LV are connected by a VSD (H–K). The location of the VSD is in the membranous portion of the interventricular septum.
Kerkela, R. (2008). Deletion of GSK-3 beta in mice leads to hypertrophic cardiomyopathy secondary to cardiomyoblast hyperproliferation. The journal of clinical investigation, 118, iss:11, 3609 -3618
Figure 2
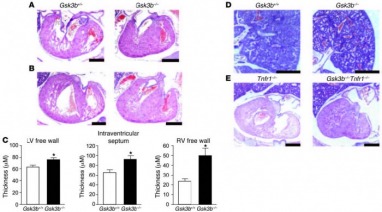
Figure 2 Analysis of ventricular development. H&E-stained sections of Gsk3b+/+ (left) and Gsk3b–/– (right) hearts (original magnification, ×2.5; scale bars: 0.1 mm) including the mitral valve leaflet (A) and the tricuspid valve leaflet (B) (original magnification, ×2.5; scale bars: 0.5 mm). The atrioventricular valve leaflets of Gsk3b–/– embryos showed cellularity, chordal attachment, and dimension comparable to those of control embryos. By comparison, the LV wall thickness was greatly increased in the Gsk3b–/– embryo hearts. (C) Quantification of thickness of the LV free wall, interventricular septum, and RV free wall in Gsk3b+/+ (n = 4) and Gsk3b–/– (n = 6) hearts. Error bars indicate mean ± SEM. *P < 0.05 versus WT. (D) The pulmonary vasculature and parenchyma showed congestion of blood in Gsk3b–/– (right) compared with Gsk3b+/+ (left) embryos (original magnification, ×1.6; scale bars: 0.5 mm). (E) TNFR1 deficiency does not rescue the cardiac phenotype of Gsk3b–/– embryo hearts. Sections of Tnfr1–/– (left) and Gsk3b–/–Tnfr1–/– (right) embryos, including the tricuspid valve leaflet (original magnification, ×1.6; scale bars: 0.5 mm).
Kerkela, R. (2008). Deletion of GSK-3 beta in mice leads to hypertrophic cardiomyopathy secondary to cardiomyoblast hyperproliferation. The journal of clinical investigation, 118, iss:11, 3609 -3618
Zebrafish – Distinct function of GSK-3α and GSK-3β during cardiogenesis[20].
Experiments with the two isoforms of GSK3 enzyme were conducted also in Zebrafish embryos. The difference of the technique was slightly different. In order to silence specifically GSK-3α or GSK-3β enzyme the scientists used Morpholinos RNAs (MO). Morpholinos are short oligo-nucleotide sequences of RNA that are complimentary to a short segment of the mRNA of the protein (most commonly overlapping the start codon) . The MO anneals to the mature mRNA of the protein in the cell and prevents the translation process, leading to lower concentration of the protein in question. The two MO use in the study were highly specific for the isoforms they were created for, and were injected in 1-cell stage embryos to determine the effect. The morphological defects were carefully recorded and showed some similarities between the two isoforms – string-like shaped heart at 72 hours post-fertilization (72hpf). After more careful examination the researchers were able to determine that the number of cardiomyocytes was decreased in GSK3 –aMO due to increased apoptosis, where as GSK3-bMO did not seem of have reduced number of cardiomyocytes but displayed defects at 36hpf and 72hpf during the looping of the heart(Figure 3). The GSK3β morphants also had some cardiac valve defects which were similar to the ones observed in axin I and apc mutants which made the researchers conclude that the cardiac valve development in zebrafish is regulated by GSK3β through the Wnt/β-catenin pathway.
The general conclusions were that 1. Both isoforms of the enzyme are necessary for the cardiac development; 2. GSK3α and not the β-isoform is needed for cardiomyocyte survival; 3. GSK3β is necessary for the determination of left-right asymmetry of the heart and its correct positioning.
As we can see there are some major differences in the results obtained from mice [2] and zebrafish [20] studies. GSK3α isoform of the mice does not seem to cause any significant defects when deleted from the genotype while the β-isoform is needed for embryo survival while both enzymes are required for normal heart development in fish. Also the studies by Kerkela [2] suggest partial redundancy in the function of the two enzymes especially in the β-catenin pathway, while the Lee’s team [18] clearly defined lack of redundancy between the two isoforms. Unfortunately β-catenin concentration and localization was not recorded in this zebrafish experiment, which I personally think needs to be addressed in order a more comprehensive analysis between the mice and fish experiment to be conducted and to determine the major differences of the GSK3 pathway in the two organisms. One additional thing that also might become a factor in this process is that the maternal effect genes involved in the process. GSK3α and β are both deposited maternally in the mature oocyte in zebrafish and silencing of the gene in the early stages can become detrimental. In mice due to the differences in embryonic development such maternal factors might be delivered to the embryo via the placenta and might be able to counteract the lack of zygotic expression of the enzyme. These are pure theoretical speculations due to the fact that my knowledge in this field is not much, but at the same time these questions can become an interesting topic for investigation in the future.
Figure 3
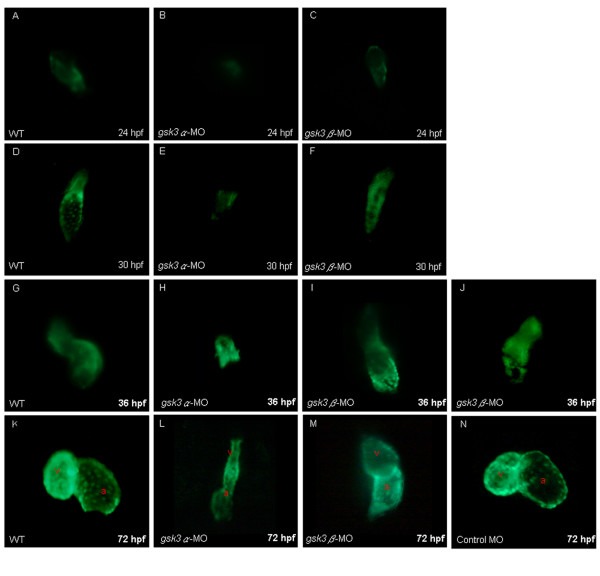
The cardiac defects induced by the knockdown of zebrafish GSK3α and GSK3β. Anti-sense morpholino oligonucleotide (MO), which was designed to specifically inhibit the translation of either gsk3α-(gsk3α-MO) or gsk3β-mRNA (gsk3β-MO), was injected into one-celled stage embryos and the heart morphology was observed at the stage as indicated. The elongation of heart tube was normally developed at 24 hpf in the wild-type (A) and in the gsk3β morphants (C); whereas the heart of gsk3α morphant did not elongate to from a heart-tube (B). The wild-type (D) and gsk3β morphant's heart (F) developed normally at 30 hpf, but the heart of gsk3α morphant was still retardant development at 30 hpf (E), and even ceased at heart-cone stage at 36 hpf (F). Compared to the wild-type (G), however, the heart positioning was abnormally in the gsk3β morphant at 36 hpf (I, J). Eventually, both gsk3α and gsk3β morphants displayed an unlooped and stretched heart (L, M). The heart morphology of embryos injected with the control MO was also observed at 72 hpf (N). a: atrium; v: ventricle.
Lee et al. BMC Developmental Biology 2007 7:93 doi:10.1186/1471-213X-7-93
Xenopus – Xgsk3 as regulator of Spemann organizer formation [22].
The studies in zebrafish suggested the involvement of the GSK3 in the Wnt/ β-catenin pathway. In order to examine the details of such control in Xenopus the researchers of this paper Pierce and Kimelman target the Lysine residue in the kinase domain, responsible for binding to ATP (see Proteomics, Structure and Function). When this residue is mutated to any other residue the ability of the enzyme to bind ATP is abolished and it cannot phosphorylate its own substrates – as the authors describe it- they created “kinase-dead mutant” – Xgsk-3K-R (Xgsk3 is the Xenopus version of the GSK3β and the K residue was mutated to R). The scientists were able to demonstrate that injection of the mutant mRNA in Xenopus embryos (especially in the ventral side) caused dorsal axis duplication (Figure 4). The same effect was observed when Xwnt 8 mRNA was injected in this spot, suggesting that the two biomolecules act on the same pathway. The mechanism by which Xgsk-3K-R acts as a dominant negative mutant was not completely clear but the most plausible explanation that the scientists provide is that the mutant protein competes with the endogenous one for substrates. The other interesting conclusion that they were able to come to is that Xgsk3 act as a repressor of dorsal fate – when the normal protein is ectopically overexpressed on the dorsal side of the embryos the resulting phenotype exhibits lack of eyes, small heads and shorter dorsal axis (results not shown); in addition the secondary axis formation was mostly observed when the mutant Xgsk3 was injected in the ventral side of the embryos, injection of the same form on the dorsal side produced over 90% normal tadpoles.
Figure 4
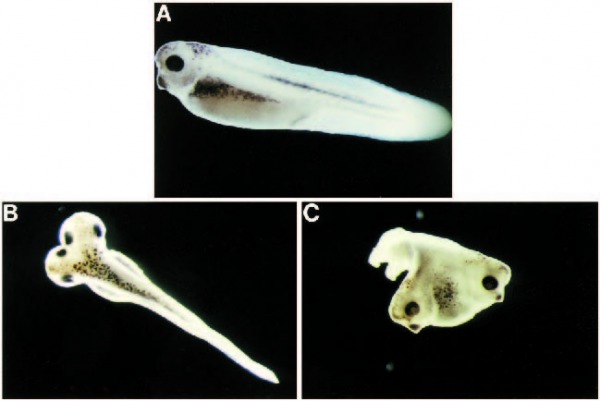
Fig. 3. Xgsk-3K®R causes dorsal axis duplication. Both cells of two-cell embryos were injected laterally with 2 ng per blastomere of DXgsk-3 RNA (A) or 0.5 ng per blastomere of Xgsk-3K®R RNA (B,C) and allowed to develop for 3 days. (B) A dorsal view and (C) a dorsoanterior view of embryos with different degrees of axis duplication.
Pierce, S. & Kimelman, D. (1995). Regulation of Spemann organizer formation by the intracellular kinase Xgsk-3. Development 121, 755-765.
Arabidopsis thaliana – GSK3/shaggy like kinase and floral cell expansion [21]
Last but not least is the homologous protein of GSK3B in plants such as Arabidopsis thaliana. In the plant there are 10 members that resemble GSK3/Shaggy-like kinases and they are known as AtSK. The difference between these members is mostly in the length of their N-terminal domain and also some members show increased level of expression in the flowers under dark conditions. In order to determine their biological roles the researchers designed different point mutations affecting key residues described in Proteomics/Structure/ Function.
The researchers mutated Lys167 and Arg178 in the AtSK3-2 isoform, which correspond to the Lys85 and Arg96 of the mammalian GSK3β. Plants which had mutation R178 (AtSK3-2 R178A) had shorter hypocotyls when grown under dark conditions (Figure 5). In addition their cotyledons opened earlier than the WT plants which can be seen as indication of defect in light-regulated processes. In addition those mutants also showed reduction in their inflorescences, flowers and siliques (Figure 6). The general conclusion that the authors come to is that these plants were defective in cell elongation.
Interestingly cells that overexpressed the mutant gene Lys 167 (AtSK3-2 K167A) showed a wild type phenotype. Consistent with mammalian studies and in vitro analysis that the authors performed previously in the experiment this mutation causes a “dead protein”. The phenotype suggests that the gene – AkSK3-2 is not essential for normal plant development. In contrast R178A mutation which is analogous to R96A in mammals causes structural changes in the positively charged pocket of the molecule and lowers the affinity of the enzyme towards primed substrates as well as prevents its ability for self-inhibition after The S residue on the N-terminal domain is phosphorylated. In other words overly active form of the kinase is created and the resulting phenotype is due to inhibition of cell elongation. The authors further investigate the topic and were able to determine that cell elongation is under the regulation of different hormone such as auxine, cytokinine, ABA and BRs. These hormones control the expression of enzymes such as XTH and XET and the expression of some XTH enzymes were down-regulated in the AkSK3-2R178A mutants which served as an explanation of the reduced organ size in the plant phenotypes.
Title Text.
Figure 5
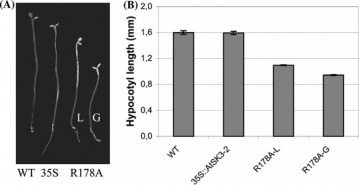
Hypocotyl elongation of the 35S::AtSK3-2-R178A mutant lines grown in darkness. WT and 35S::AtSK3-2 plants (35S), as well as two 35S::AtSK3-2- R178A (R178A) independent lines (L and G) were submitted to darkness (A),and their hypocotyls weremeasured (B) (n = 20–40).SDs are displayed
Claisse, G. & Charrier, B. (February, 15 2007). The Arabidopsis thaliana GSK3/Shaggy like kinase AtSK3-2 modulates floral cell expansion. Plant Mol Biol 64:113-124.
Figure 6
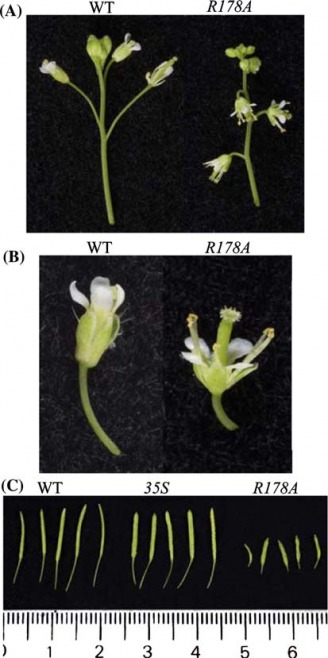
Flower morphogenesis in the 35S::AtSK3-2-R178A mutantlines. Size of inflorescences (A), flowers (B) and siliques (C)in wild-type, 35S::AtSK3-2 (35S) and 35S::AtSK3-2-R178Aexpressing plants. Scale in cm
Claisse, G. & Charrier, B. (February, 15 2007). The Arabidopsis thaliana GSK3/Shaggy like kinase AtSK3-2 modulates floral cell expansion. Plant Mol Biol 64:113-124.
References
2. Kerkela, R. (2008). Deletion of GSK-3 beta in mice leads to hypertrophic cardiomyopathy secondary to cardiomyoblast hyperproliferation. The journal of clinical investigation, 118, iss:11, 3609 -3618
19.Doble, B. & Woodgett, J. (2003). GSK-3: tricks of the trade for multi-tasking kinase. Journal of cell science 116, 1175-1186
20.Lee, H. & Tsai, J. (August, 3 2007). Glycogen synthase kinase 3α and 3β have distinct functioning during cardiogenesis of Zebrafish embryo. BMC developmental biology, 7:93.
21. Claisse, G. & Charrier, B. (February, 15 2007). The Arabidopsis thaliana GSK3/Shaggy like kinase AtSK3-2 modulates floral cell expansion. Plant Mol Biol 64:113-124.
22. Pierce, S. & Kimelman, D. (1995). Regulation of Spemann organizer formation by the intracellular kinase Xgsk-3. Development 121, 755-765.
23. Dajani,R. (2001). Crystal structure of glycogen synthase kinase 3 beta: structural basis for phosphate-primed substrate specificity and autoinhibition. Cell 105, 721-732.
Back to: Home
Contact Information
Eva Dimitrova
[email protected]